The most important aspects of my doctoral research are summarized here. My dissertation was on the kinematics and the dynamics of a model eastern-boundary poleward undercurrent. Kinematics of fluid motion is a description of the appearance of flow, without consideration to forcing mechanisms. Dynamics focuses on the physical mechanisms that drive the flow. Thus, kinematics is a proper place to begin the study of the dynamics of fluid motion. Can the dynamics of fluid motion be studied correctly, if the motion itself is not well understood?
My dissertation is the first analysis of a carefully calibrated primitive-equation numerical ocean model designed to study the undercurrent. Understanding the dynamics driving the undercurrent has been challenging because many analytic and numerical models produce undercurrents, while arriving at a diverse set of conclusions, some of them contradicting. Thus, over a century after a first paper on poleward flow off the U.S. west coast was written, the dynamics of the undercurrent remains an open question. In my dissertation, I was able to show that the dynamics of the undercurrent within a modern ocean model are consistent with the analytic theory proposed in the 1970s by one of the greatest oceanographers, Joe Pedlosky, and later endorsed by another of the greatest oceanographers, Gabriel Csanady. Ongoing work with my Ph.D. adviser, Roger Samelson, is testing this hypothesis further. The problem of extracting the dynamics from the primitive-equation model was challenging because, as it turns out, the balance of interest is overwhelmed by energetic coastal waves.
Kinematics
Comparing our model results with a large number of observational and modeling studies resulted in a robust depiction of an eastern-boundary undercurrent. However, this depiction is not the typical one in the literature, despite the supporting evidence spanning over six decades of scientific studies. In my view, this is an example of how incomplete measurements skewed our collective understanding. For example, the following three characteristics are the norm in observational studies that measure deep enough, and also in primitive-equation modeling studies in general. In eastern-boundary systems:
- Poleward flow over the slope consistently reaches 1–1.5 km deep.
- There is poleward flow over the slope year-round, albeit deeper than ~400m in spring.
- During upwelling months, poleward flow over the slope reaches the surface, unless there is a coastal jet in which case there is a proper undercurrent.
In contrast, a typical description would say something more like: “A persistent and fast (peak velocity 20-50 cm/s) subsurface poleward current (the California Undercurrent) flows along the upper continental slope and outermost shelf between about 150-300 m depth.”, and “This undercurrent is absent immediately after the spring transition which marks the onset of seasonal upwelling … but it appears within a few weeks and slowly intensifies through the summer.”
Of the three characteristics listed above, the third is relevant to my results on the undercurrent dynamics, I, therefore, discuss this below. Those interested in points 1 and 2 above are encouraged to read the discussion of chapter two in my dissertation (section 2.6).
Poleward flow over the slope tends to reach the surface during upwelling.
It is well known that in the winter, poleward flow over the slope in eastern boundaries reaches the surface. Less appreciated, however, is that poleward flow also tends to reach the surface during the upwelling season, whenever there isn’t an upwelling coastal jet. In the winter, poleward flow is surface intensified, while during upwelling season, poleward flow reaching the surface is bottom intensified. The model simulation does a good job of replicating this feature, e.g. monthly Eulerian-mean meridional velocity (m/s) plotted at 42^\circN as a function of depth and longitude, showing surface intensified poleward flow reaching the surface in January, October, November, and December, and bottom-intensified poleward flow reaching the surface in July through September:
The reason poleward flow reaches the surface during July–September in this cross-section, is because the coastal jet has separated just north near 43^\circN; the coastal jet can be seen, e.g., at 125.5^\circW in July. The coastal upwelling jet tends to separate near Cape Blanco (42.7^\circN).
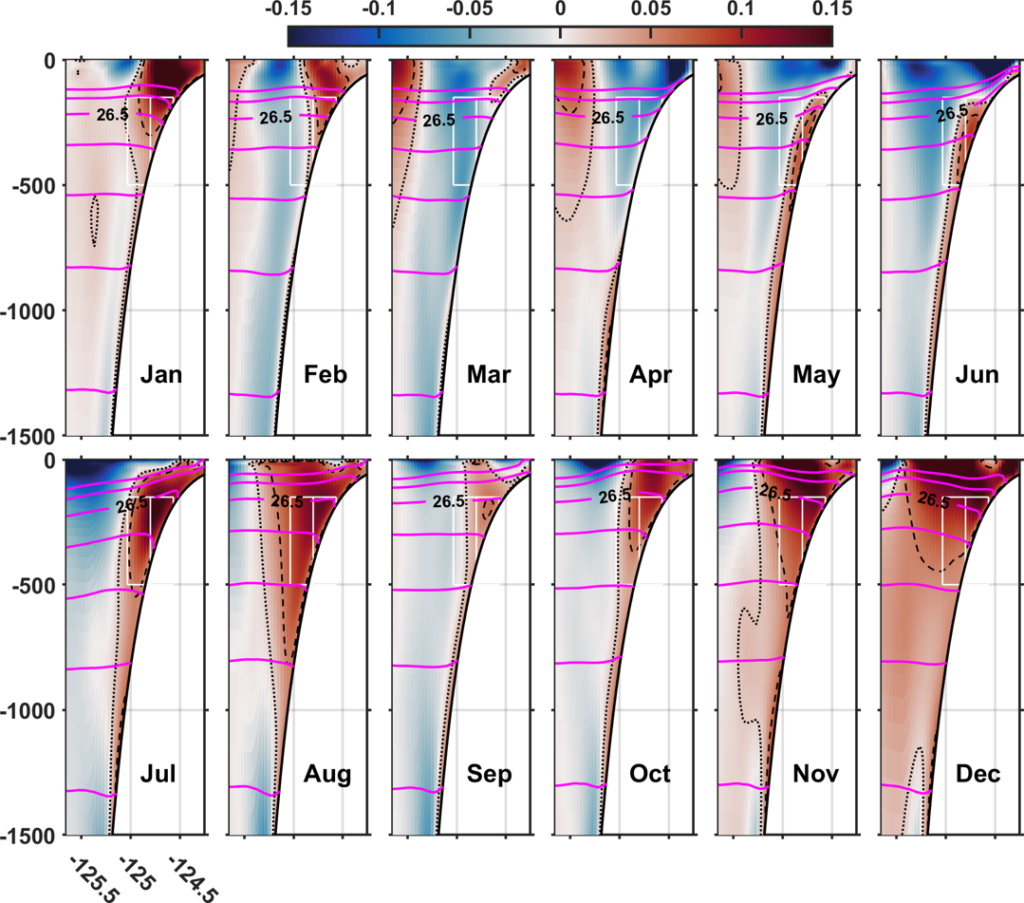
ADCP sections taken in 1995 near Cape Blanco off the coast of Oregon and analyzed by Barth et al. (2000), capture the coastal jet separation and its interaction with the undercurrent. Note how poleward flow reaches the surface just west of the jet and is bottom intensified, this is before the coastal jet has separated (43.22^\circN):
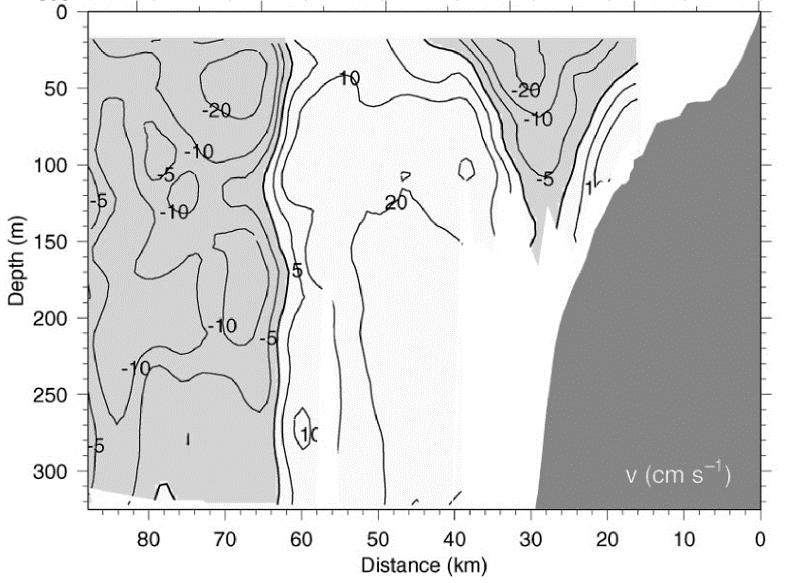
Once the jet has separated (41.9^\circN), the bottom-intensified poleward flow reaches the surface over the slope, while the coastal jet is further offshore:
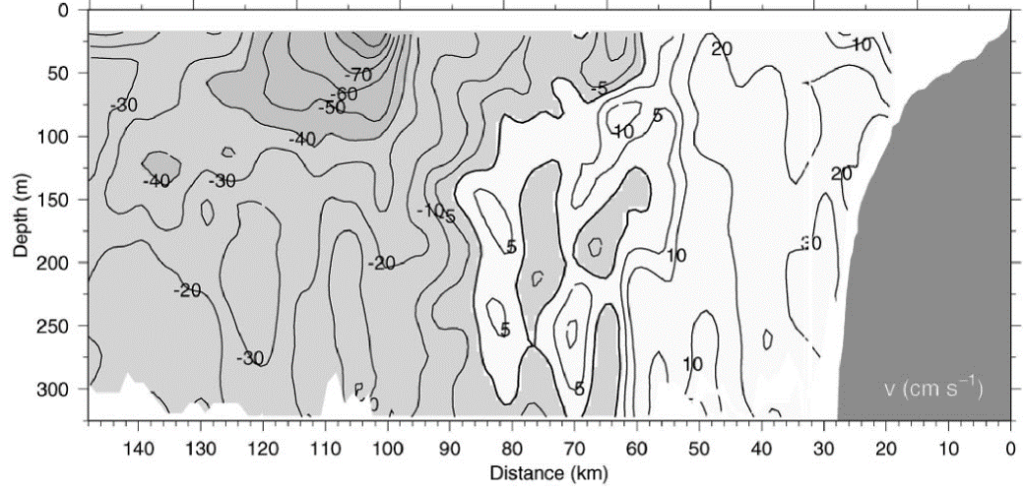
A sequence of three snapshots off the coast of northwest Africa (Barton et al. 1977) clearly illustrates the interaction between an accelerating coastal jet and poleward flow over the slope. From left to right, in the first snapshot poleward flow reaches the surface over the slope and the coastal jet is confined inshore. As the coastal jet accelerates, it increases in offshore extent (middle panel), and poleward flow becomes an undercurrent. The panel on the right shows an even stronger coastal jet with a poleward undercurrent beneath it—this is what is typically presented as an eastern-boundary poleward undercurrent:
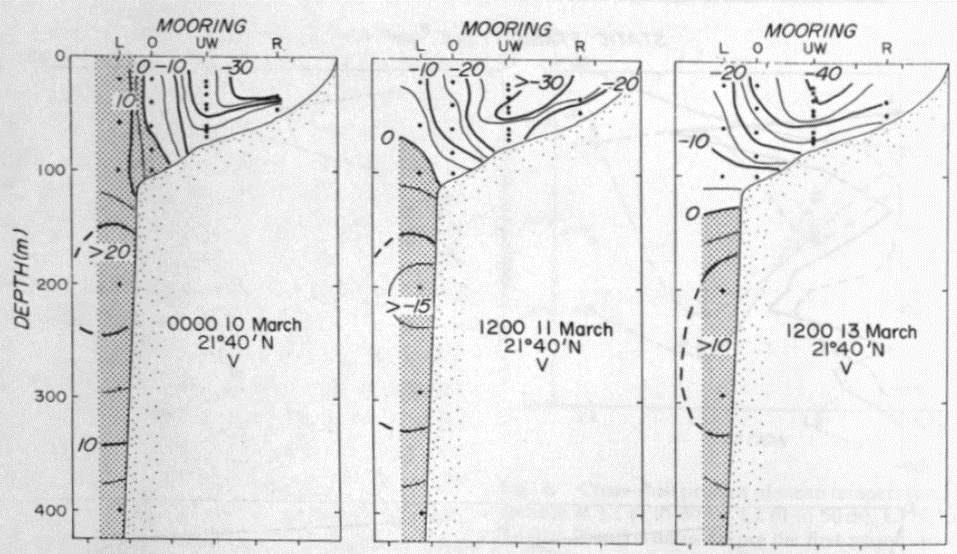
Many more studies, observational and modeling, support that poleward flow over the slope typically reaches the surface during upwelling season except when and where there is a coastal jet, references can be found in the discussion of chapter two of my dissertation. Here I present one more example from Huyer et al. (2007)—a seven-summer (1997–2003) mean of meridional velocity from ADCP measurements off the coast of Oregon. Notice how poleward flow reaches the surface just offshore from a coastal jet that has not separated (this section is at 44.65^\circN), there is a poleward undercurrent just below the jet:
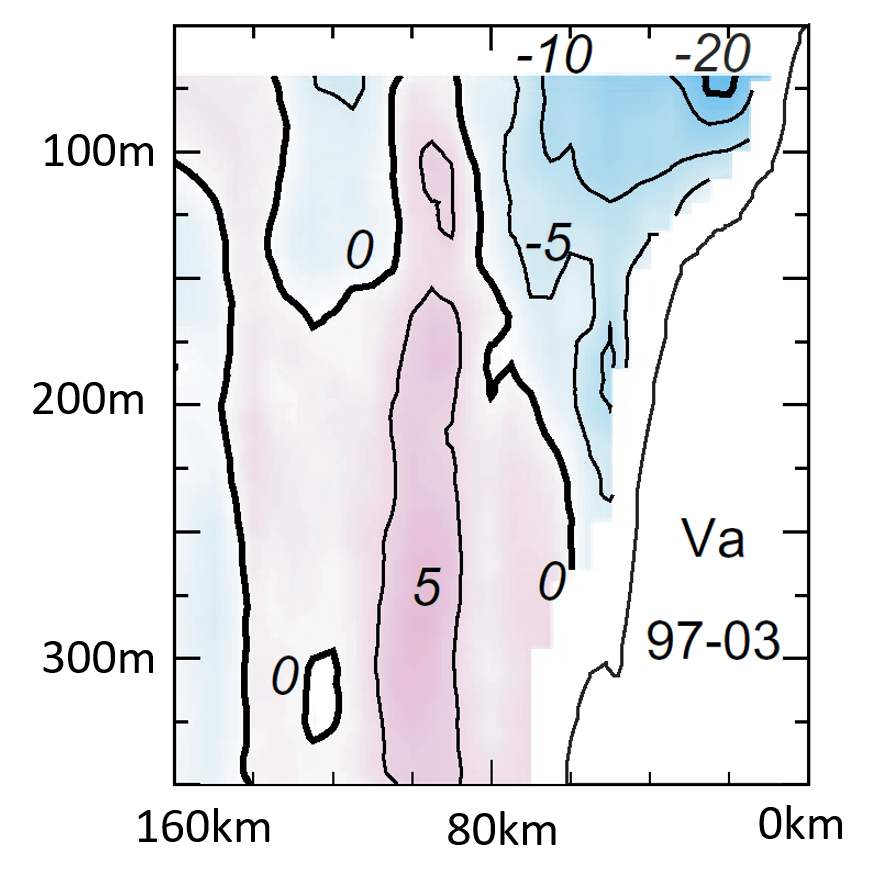
The following movie from the simulation I studied shows the interaction between poleward flow and the coastal jet. It is the coastal circulation from June through December 2005, off the coast of Oregon and Washington (41–48^\circN). The coast is shown in black, the day number is shown above, and the month is shown below. The cyan isosurface is given by v=-8cm/s, the magenta isosurface is given by v=+8cm/s, where v is the meridional velocity.
In June, surface coastal circulation is equatorward (cyan) through the meridional extent of the domain, with a poleward undercurrent over the slope. Starting in July, the coastal jet beings to separate near 43^\circN (Cape Blanco), and poleward flow often reaches the surface to the south of 43^\circN once there is no coastal jet above it. When the poleward flow reaches the surface south of 43^\circN there is a competition of effects, wind is upwelling favorable through the domain. There is an undercurrent below the coastal jet north of 43^\circN. This continues to be the case through the rest of the upwelling months. In early October a very clear coastal jet can be seen separating near Cape Blanco, with a clear undercurrent north of 43^\circN and poleward flow reaching to surface south of 43^\circN.
To be continued, this is work in progress…
Continue here …
References
- Barth, J. a., S. D. Pierce, and R. L. Smith (2000), A separating coastal upwelling jet at Cape Blanco, Oregon and its connection to the California Current System, Deep Sea Research Part II: Topical Studies in Oceanography, 47(5-6), 783–810, doi:10.1016/S0967-0645(99)00127-7.
- Barton, E. D., A. Huyer, and R. L. Smith (1977), Temporal variation observed in the hydrographic regime near Cabo Corveiro in the northwest African upwelling region, February to April 1974, Deep-Sea Research, doi:10.1016/0146-6291(77)90537-9.
- Huyer, A., P. A. Wheeler, P. T. Strub, R. L. Smith, R. Letelier, and P. M. Kosro (2007), The Newport line off Oregon – Studies in the North East Pacic, Progress in Oceanography, doi:10.1016/j.pocean.2007.08.003.